Steve W. McLaughlin
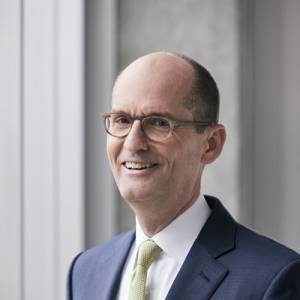
Anna Erickson
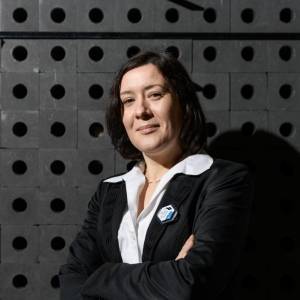
Audio
Audio & Captions
Transcript
[steam whistle]
[“Ramblin’ Wreck from Georgia Tech”]
>> I'm Steve McLaughlin, dean of the Georgia Tech College of Engineering, and this is The Uncommon Engineer.
Archived recording: We're just absolutely pleased as punk to have you with us. Please say a few words.
[applause]
[music]
Archived recording: But the process of making electricity with nuclear fuel takes place behind protective walls of concrete and steel and, therefore, can't be seen. What we can't see we sometimes don't understand until we look from a different perspective.
Steve McLaughlin: The 2019 award-winning HBO series Chernobyl tells the story of the 1986 nuclear plant explosion, which took the lives of 28 workers and inflicted radiation symptoms on approximately 3.5 million people.
Nuclear energy is a powerful proposition that can create clean energy for our planet. But as the landscape for nuclear power and the proliferation of nuclear technology and the threat of nuclear war remain constant, the public remains worried.
Welcome to another episode of The Uncommon Engineer podcast. I'm Steve McLaughlin, dean of the Georgia Tech College of Engineering. The Uncommon Engineer discusses how Georgia Tech engineers make a difference in our world, in our daily lives, in ways you might not expect.
Our guest today is Doctor Anna Erickson. She's a professor of nuclear and radiological engineering within our Woodruff School of Mechanical Engineering here at Georgia Tech. And she's an expert on nuclear security. Welcome to the program, Anna.
Anna Erickson: Thank you for inviting me, Steve.
Steve McLaughlin: It's nice to have you here today. We're going to talk about nuclear power, nuclear safety. I think almost everybody is—when we think of nuclear power and safety, we think of things like Chernobyl and Three Mile Island, you know, things that happened quite a while ago. A lot's happened between now and then. I think we're could talk about that. But can you just start by talking about some of the disasters that have occurred? What technology is involved, maybe why some of them occurred, and then we'll get to where things stand today.
Anna Erickson: Well, this is a great question and I think you're captured that precisely, that disastrous is what became that defining moment when we talk about nuclear power, which is both frightening and unfortunate for us as nuclear engineers.
So let me start perhaps with that recently released HBO series, mini-series, Chernobyl. It is interesting that, historically, there was not a lot of information about Chernobyl because of Soviet Union and how closed off information was. And that mini-series tried to really fill that gap, the vacuum of information we had.
And it did two things. It really allowed us to see why disasters like that could happen. And in part, that was the Soviet Union culture to blame and also the effects of the nuclear disasters. Nuclear disasters is what gives the nuclear power the negative attitude from public. And this is something that we consistently had to battle in our field.
Steve McLaughlin: Between the time of those disasters and now, you know, the world has become much more aware of the effect of hydrocarbons, you know, on the atmosphere and the environment. And so it seems that nuclear power has become more acceptable. It's viewed now in a more positive light as a clean energy source. And I think the reputation around nuclear energy… But as we said, you know, I think people still have those disasters in the mind, but I think the reputation of nuclear energy has impressed—has improved a great deal.
And so can you talk about maybe how that's happened? And also you know what… What's going to happen in the next 10 years for nuclear power?
Anne Erickson: Well, you are spot on there, that in the past two years, the reputation of nuclear energy is changing. And I think the fundamental reason is the existential threat that we face right now as humanity of global climate change and the battle with the hydrocarbon sources of energy.
Now, I think this is common, that for something to change your reputation, you need to have some sort of existential threat associated with it. And we experience that now. So one example I can give you, a couple rather, what's going on in the United States is the construction of Vogtle power plant, which of course has been going on for a few years, but it's here in Georgia. It's local. It's happening and we're hoping the construction will wrap up soon and we'll be able to use the nuclear power.
And then the second is a little more exotic example. It's called “versatile test reactor.” And this is something the Department of Energy initiated a few years ago to create a new generation reactor to show the benefits of not only the traditional technologies but also technologies of the future. Of course, as I may preempt the question that might follow, how do we safeguard the technologies of the future? How do we make them safe and proliferation-resistant, still remains a big question to answer.
Steve McLaughlin: I'm assuming safety has improved, but maybe safety has been there all along and we just haven't really appreciated it. Right? Nuclear power plants are still out there. Tremendous amount of power is being generated by it. We kind of have forgotten maybe how safe nuclear power really is. And so is that a true statement?
Anne Erickson: This is a very true statement. And I thank you for bringing this up. And I want to point out that there's a difference between safety and proliferation, right? So safety is related to how well can you run the reactor safe, meaning not affecting the public health or the reactor health.
And if I may highlight the fundamental difference between the current reactors in the United States, which we have 98 as of this January I believe, generating about 20 percent of our electricity versus what happened in Chernobyl. And allow me to circle back to that design again. Chernobyl design was not light water reactor based. It was designed with a dual purpose in mind: to produce clean plutonium for weapons program and generate electricity for a civilian program. Now, we all know after the 1986 Chernobyl disaster what happens when you mix the two purposes. And that reactor, from a safety standpoint, was very different. It had what we call “positive reactivity coefficient.” And I'll explain what that means. What that means is, as the reactor gets hotter and hotter, the neutrons that you produce the reactions—the main driver of the reaction becomes more and more intense. So instead of shutting down because it's getting hotter and less safe, it actually instigates to further reactions.
Light water reactors here in the United States operate on the different principle. What happens when you heat up the water? It expands because its temperature goes up, density goes down. When the density goes down, the neutrons don't become as active. So you actually shut down the reactor as you're getting it hotter. And that is the fundamental principle of design features of reactors here. We make them safe and safer as they get out of control.
So that doesn't mean that accidents cannot happen. But we design those systems with such safety coefficient in mind. I think it's safe to say that nuclear reactors have one of the safest records among many industries in the States.
Steve McLaughlin: And so I know that you're interested—so I'd really like to talk about the research that's going on in your group and some of the projects that you're involved in. And so we've talked a little bit about nuclear safety. Can you say some things about your work, the work of your students and colleagues around nuclear safety?
Anna Erickson: This is a great question. The name of our research group is Laboratory for Advanced Nuclear Nonproliferation on Safety, so you see there are two key words there: nonproliferation and safety. So far we've discussed safety, so if you don't mind, I say a few words about nonproliferation and what that means.
Nonproliferation is limiting the spread of nuclear material and nuclear weapons and limiting the effects around the world. So my group is mostly focused on bridging that gap between production of nuclear material and disposition of nuclear material and every step in between and ensuring that every step is both safe and secure from a proliferation standpoint. So we are interested in every subcategory of that. We are interested in the reactor design and ensuring that they're inherently safer and proliferation-resistant. And my recent paper on antineutrino monitors is a highlight of that. And we’re also interested in once the nuclear weapon is out there, how do we find it? And how do we pinpoint who might have done it? So from a nonproliferation forensic standpoint.
We were proud to get awarded a new grant from the National Nuclear Security Administration, which is part of Department of Energy, and the new initiative is called Consortium for Enabling Technologies and Innovation. And this is a very large group of people. We have 12 universities and 10 national laboratories who are interested in understanding how we can create technologies of tomorrow to solve the proliferation problems that may not even be known to exist yet.
And I'll give you some examples. For example, one of our initiatives is to look at advanced manufacturing. Why would we care about that? Well, the proliferation, if I may use that word, of additive manufacturing technologies is so widespread today, we don't know who may be using it and for what purposes. There are some conceptual designs of microreactors and mini-reactors that are floating around. So how do we safeguard those future technologies from falling into the wrong hands? And how do we predict what kind of problems we may anticipate in the future?
Another example is artificial intelligence. Machine learning, in particular, has been receiving a lot of attention. We see it on every corner of every discipline. In no different than other disciplines we are interested in both leveraging the machine learning technologies to our advantage and also to understand how it can be used by our adversaries to create and proliferate nuclear weapons.
So let me wrap up with the saying that we have three thrust, a total of three thrust in that consortium. The third one is investment in novel nuclear instrumentation, meaning not the instruments that have been around since 1950s but something that's fundamentally different, some materials that have not existed 10 years ago. Now we are trying to tailor them for detection of radiation.
Steve McLaughlin: I kind of have two questions. One is I'm curious to learn a little bit more about how artificial intelligence connects with nuclear safety or nuclear reactors. And second is a lot of things that come about in artificial intelligence really worry me, because they can be used for good, but not all our algorithms are accurate and not all algorithms make the right decisions. And so do you want to talk a little bit about the artificial intelligence space and what you're working on?
Anna Erickson: Well, that's a great question. I should make a disclaimer that I'm not an expert on artificial intelligence and machine learning, but I rather work with the experts to make sure that we connect them with the problem of nuclear nonproliferation. And in particular, there are a few different instances where machine learning becomes quite useful. Think about a distributed network of sensors around the globe. Maybe you’re monitoring radio-xenon to make sure that there's no nuclear explosion that's happening. How do you share that information in the most efficient way? How do you ensure that hundreds of sensors communicate and make a decision globally, as opposed to drawing data from each individual sensor and then report it to a human operator?
So this is where we see machine learning being the most useful, as far as application to radiation detectors, analyzing hundreds of data streams simultaneously, drawing patterns and drawing conclusions that would not be possible otherwise. And also for monitoring patterns and changes in patterns from day to day, month to month to ensure that we have the maximum information.
Another example of machine learning is application to the systems that are mobile. For example, how do you make a swarm of robots work together, especially when they are outfitted with radiation sensors and they collect data from various points spatially and temporally? How do you coordinate them? How do you know where to look for a potential threat? So all these questions are really difficult to answer without artificial intelligence and machine learning in nonproliferation activities.
Steve McLaughlin: The team that you described as being very, very diverse is so incredibly important for A.I. like systems to work properly. We just have to have the maximum number of diverse perspectives, whether scientific, whether it's gender, whether it's ethnic. All of those perspectives need to come to the table, because if we don't have all those perspectives, we don't consider all those possibilities. And so that's seems to be one of the real powers of your team, as you describe, this really diverse set of folks who don't work together and so on. You make me more optimistic, not just about your project, but about all projects, you know, that we need to keep that in mind. Was that one of your goals?
Anna Erickson: Yes, I think that's a pretty good description. And in particular, any discipline is a black box to each other, right? If you think of radiation detector, it's probably a black box. It may be making noise or it may be telling you some numbers, but what does it really mean? Where's the threat? Where is the good operating point for nuclear reactor if this is a sensor associated with the safety?
You need people to come together and work on the problems in order to get the best benefit. Before I started working on our consortium, artificial intelligence was nothing but a black box to me. It was a set of algorithms or methods that we could use to do X, Y, Z. But once you start working with the experts, we can really power up the cause and effect of those algorithms, because we know what applications we could use them the best and they know how to implement them the best.
Same goes for pretty much every engineering discipline. That's why being part of the engineering team at Georgia Tech is fascinating, because we have five different faculty from this campus, including aerospace, materials science, E.C., electrical and computer engineering, nuclear engineering, and of course mechanical engineering working together to tackle those uncommon problems.
Steve McLaughlin: Well, you know, it seems impossible to talk about nuclear power without talking about global, what it means internationally and whether it's the collaboration, whether it's the technologies, whether it's the use and policies. Can you talk a little bit about nuclear power and its international aspects and where that fits in with your work?
Anna Erickson: That's a great question. Let me start with the quote that is familiar to all engineers because we are geeks, right? So with great power comes great responsibility. This is an excellent representation of nuclear power. Remember how nuclear power started in 1945 with the deployment of a nuclear weapon. But what it's evolved into is with the help of President Eisenhower and his program Atoms for Peace, it evolved into peaceful use of nuclear energy that we harvest today. And why would the US be the only one to use it? There are multiple countries around the world that want to exploit the positive effects of nuclear energy.
And there are two questions, of course, that are always on the mind: Are they doing it for peaceful purposes? And if you suspect it's not being used for peaceful purposes, what do you do about that and how do you monitor for that?
So it's actually interesting that a few days ago, we celebrated 62nd anniversary of International Atomic Energy Agency, which is the main body that allows us to monitor what happens around the world at the nuclear power plants and to ensure that the nuclear material is being used for peaceful purposes. So the IAEA has been established as a result of the global Atoms for Peace program. It was inaugurated by President Eisenhower. And to this day we use it to our benefit.
So how do we know that nuclear power is being used for peaceful purposes? The agency sends its agents around the world and they inspect nuclear power plants, sometimes periodically and sometimes unannounced, and they ensure that nothing nefarious is happening at the reactor sites and other facilities associated with nuclear materials.
Unfortunately, there are certain states that are not signatories to Nuclear Proliferation Treaty, which is what allows those inspectors to go around the power plants. Some of the examples include well-known North Korea who withdrew from the Treaty in 2003.
Steve McLaughlin: So in places like North Korea where the inspectors can't show up, what do you do?
Anna Erickson: This is a great question, and I wish I had all the answers. But it's a very valid question, and not just with respect to North Korea, but other plants as well. The inspectors are not present there 24/7. So how do you ensure that things run continuously? And there are a number of things that have been implemented over the years: seals and detectors associated with IAEA that continuously read out the data from reactor operations. But one of the more novel technologies that my group in particular is working on is antineutrino monitoring devices. I think I mentioned earlier that we just had a paper came out in Nature Communications with an intent to show people how those antineutrino devices can be used to monitor the reactor continuously, even when the inspectors are not on the ground.
Steve McLaughlin: And so say more about the antineutrino devices and how they fit in.
Anna Erickson: So every reactor emits copious amounts of antineutrinos. It's just a byproduct of nuclear fission reaction. So we can correlate antineutrinos with the number and types of fissions. Hence, we can monitor for both the evolution of the fuel in the core and any type of tampering with the core. Of course it has its limitations and, in particular, the amount of material that people tamper with could be limited.
But you brought up a good point: How do you do it remotely? So antineutrinos are such small particles, you cannot shield them. That means that they can travel very, very far distances. In fact, there are millions and hundreds of millions of antineutrinos going through your body right now and you have no idea that it's happening. The entire Earth to antineutrinos is nothing. They don't even notice that they exist. They just go through. So we can exploit that to our effect. We can build very large detectors and place them far away from nuclear reactors. And there are a number of projects going on around the country. And one notable example is the WATCHMAN Project, which is led by the Lawrence Livermore National Laboratory. But you can also think about positioning those detectors near a nuclear reactor but outside perhaps the boundary of the reactor and really get the maximum information from the nuclear fuel cycle.
Now, I should point out that nuclear radiation, the traditional radiation in the form of neutrons and gamma rays, that can be shielded quite easily. So satellites will be oblivious to nuclear radiation, but other technologies can be exploited to monitor those reactors.
As far as radiation detection technologies, we work on a number of different techniques. So one of our concerns, of course, is making sure that there are eyes on the reactor at all times, so boots on the ground, right? So antineutrino monitor is a good example of that. You cannot change the signature of nuclear reactor in the form of neutrinos. The only way to do it is to build another nuclear reactor right next to it. So if there is tampering with nuclear fuel, we should really know that, we will take note of that using the antineutrino monitor. So that's one aspect of radiation detectors that we work on, the technology that's relatively exotic.
The other aspect is how do you track the movement of nuclear materials? I'm shifting gears a bit here from monitoring the reactors to monitoring the material. And one big area of concern is from Homeland Security, right, Department of Homeland Security. Their particular concern is 40-foot containers crossing the country's boundaries and arriving by sea. How do you know what's going on inside of those containers? And keep in mind that those containers arrive to the busiest sea ports in the country, such as Los Angeles and New York. So if something devastating were to happen in one of these containers, we would feel the effect.
So the Department of Homeland Security is concerned with uncovering special nuclear materials while it's en route to the country, and a big part of my research is to build systems that allow us to sense those type of threats inside of large objects like containers. We call it the active interrogation method for nuclear material. And last year in 2018, me and a colleague of mine from University of Michigan, Igor Jovanovic, we published the very first book on using active interrogation for nuclear security applications and all the details of the technology that goes in there.
Steve McLaughlin: I'm really curious how you do that.
Anna Erickson: It's almost mind-boggling because we have a dual problem, triple problem I suppose. First we need to find the material. But second, we must not slow down the movement of the containers. The Port Authority would really not allow you to do it. So you have about 40 seconds to two minutes to inspect a 40-foot container. That's not a lot of time to find anything.
And then the third problem is the responsible use of radiation when you do that. We know examples of human stowaways in containers, and the last thing we want to do is to hurt a human being that may be present, or to hurt a human operator that's operating our machines. So we have to combat this a triple-pronged problem and to be effective at doing so.
So typically, what we do is we use an external source of radiation, just like x rays in the dentist office, to what we call “interrogate” an object, for example a container. And on the other side of the radiation source, we have a set of detectors that will read out what happens inside of the container. So our problem is to not only create detectors that are capable of reading out fast and recreating the objects but also create a set of algorithms that allows us to reconstruct what's inside.
Steve McLaughlin: You know, if there's a technology or area that generates all kinds of really strong opinions, positive and negatives, you're saving humanity, you're killing humanity. You know, nuclear energy, nuclear power, nuclear weapons, nuclear nonproliferation, all of those. You're right in the middle of all that. So how did you decide that that was the area you wanted to study and make your life's work about it?
Anna Erickson: Well, first of all, my life is never boring. Thank you to the field that chose me. So I think the question is how did I decide? I think that was decided for me, because I saw no other field that would be so interesting and so diverse in its application.
I still remember reading about Chernobyl when I was a kid. This was a fascinating area for me from childhood. And I know how cliché that sounds, but it's so true that nuclear technology is one of the most diverse and controversial subjects. And just think of the breadth of that field from we started as a nuclear weapon field, right? That's the inception point. But today, the human lives that they saved because of radiation cannot be counted. A recent example is Proton Center right here at Emory that is designed to save human lives and to make the treatment much safer than it could be with other technologies. So I think being right in the middle of that and trying to take the best out of this technology for the humanity is what makes my day the most exciting.
And as far as the research and my students, we cover a broad scope of students. I have medical physics students as well as students working on nonproliferation issues. And you know what unites us? We have a common goal. We want to use the radiation for the best of humanity and we want to ensure that when the technology falls in the wrong hands, we know about that and we want to prevent that. And that goes from creating nuclear materials all the way to medical products, for example accelerators and technologies related to that.
Steve McLaughlin: Well, one of the questions we always ask our guests on The Uncommon Engineer is what makes you an uncommon engineer?
Anna Erickson: Well, I think we covered that.
Steve McLaughlin: I think we did.
Anna Erickson: Let me point out that the uncommon part of being a nuclear engineer is that we can't do the problem ourselves. We interact with every kinds of engineering to make sure that we solve the problems that may seem common on the surface, but they have really great defects.
I started with the quote of “With great power comes great responsibility,” and I'd like to end with that as well, because this is so true in our profession. What's uncommon is the amount of energy and the impact that we deal with on everyday bases and being able to tame that impact and knowing the effects and being able to control it is what makes us uncommon.
Steve McLaughlin: Well, I can't tell you—I'll thank you on behalf of all of humanity for that, because we need people who are willing to tackle the most challenging and most difficult, both scientific as well as signed a societal, problems. We're lucky to have you here, we're lucky you came today and that you do the work that you do and that you are… I really love that perspective of, for now the third time, “With great power comes great responsibility,” and it sounds like you take both of those extraordinarily seriously and we're really thankful to have you here and thank you for everything you do for our students and for Georgia Tech. So thanks for coming today.
Anna Erickson: Thank you for inviting me. And I want to highlight that Georgia Tech is a wonderful place to be a nuclear engineer, and I think we have one of the top student cohorts among all universities and I'm very proud to be a Yellow Jacket.
[“Ramblin’ Wreck from Georgia Tech”]
Geekout
Audio & Captions
Transcript
[scanning radio station dial]
[big band swing music]
Steve McLaughlin: —sounds incredibly complex—it sounds like—you need to have abilities that span—I’m really geekin’ out here.
[laughter]
[big band swing music]
[ominous music, Geiger counter]
[archival recording] Announcer: One of the greatest energy discoveries—nuclear energy.
Steve McLaughlin: So one of the things that you mentioned was around advanced manufacturing, and so let me see if I have that right. So I think a lot of people are familiar with 3D printers, you know, the ability to, on your own desktop, you know, build and construct things as long as you have a computer and you can connect your computer to a 3D printer, you can produce or make almost anything possible. I think you're saying, you know, more advanced versions of 3D printers can be used to manufacture weapons or manufacture—and you also mentioned micro-reactors. And so people, our adversaries, are thinking of using some of these advanced 3D printing-like technologies to make their own reactors. Is that right?
Anna Erickson: That is actually getting there. I would say people are probably not there yet to manufacture a weapon, but some recent examples of people 3D-printing guns or even a whole car. Oak Ridge news was released a few months ago that they 3D-printed vehicle. It's not difficult to extrapolate that you can use the new advanced technology to 3D-print components and systems that can be used for nefarious reasons. And another example is Oak Ridge, as well, reported that they 3D-printed a mini-reactor using nothing but 3D printers. So the technology is out there. We got the genie out of the bottle. Now we need to know how to control it and be able to understand whether the technology is used for peaceful purposes or something else.
Steve McLaughlin: So how do you do that? How do you—how would you build a 3D printer or how would you—what kind of design methodologies or thinking go into, say the manufacture of 3D printers so that it's not used for nefarious purposes? Part of me is thinking “How in the world can you prevent that?”
Anna Erickson: Well, it's the same way we’re preventing nuclear material from being misused from reactors. We monitor closely and we create sensors and technologies that they are able to tell us what's going on inside of that 3D printer. So not going into too many details on the manufacturing side, I can tell you that we have leading experts in both advanced manufacturing and maker’s communities and 3D printing to help us understand the problem. So what makes our consortium unique is that expertise from people that almost never work together. How often do you see advanced manufacturing person working with machine learning or radiation detection people? Almost never. So this consortium gave us an ability to make connections between communities that that not typically involved in the problem of nuclear nonproliferation, but they're now together to solve it.
Steve McLaughlin: You mentioned a couple examples. So you want to share a couple of those examples of inaccuracies?
Anna Erickson: Well, this is a great setting to discuss that because what is nuclear energy? What is radiation? When I ask people, they struggle to answer that. We are not born being able to sense radiation. This is what's different about it: You can't touch it or see it. And this is the frightening part because you don't know when you're exposed to radiation and you don't know what effects might happen now or 30 years from now. So a couple of inaccuracies—I would call them anecdotal, of course, they were sad at the end—interesting story was told about a woman who was pregnant and who spent a lot of time near her dying husband. He helped to clean up, or to mitigate the disaster after Chernobyl happened. And she was pregnant and, unfortunately, her baby died a few days after it was born. And the HBO series portrays it as the baby absorbed the radiation and saved the woman, but the baby died. There's really no such thing as absorbing the radiation by one human being to save another. Radiation affects everybody and you can think about small humans, fetus in particular, is a lot more susceptible to radiation effects.
And other inaccuracies: When people were exposed to radiation and were done in hospitals, it was portrayed as they could irradiate other people. Now, just because you're exposed to radiation does not mean you become radioactive. It is not practically possible unless the radiation source got inside of your body and remained there. An example would be nuclear medicine treatment: When you ingest a source and it stays within your body for some period of time, now you became the radioactive source and could irradiate other people perhaps.
So some inaccuracies like that that highlight the importance of education of the public about nuclear energy, nuclear sources, and radiation in general, that could perhaps help alleviate that fear of radiation—fear of unknown and invisible.
Steve McLaughlin: What I'm really curious about is if almost nothing—an antineutrino can pass through anything, so they’re—anything that it passes through is totally transparent, so how do you detect it? You know, what’s—because any kind of detector, the antineutrinos are going to pass through don't even see it, but yet it still must be detectable. So how do you do that?
Anna Erickson: Well, this is a great question. It has to do with not only how you detect that—the detection method is actually relatively straightforward, well, for nuclear physicists, I guess—when antineutrino interacts with the proton, which is what hydrogen is made out of, right—nucleus of hydrogen is a proton—it turns that proton into two particles that we are very familiar how to detect. It's called the positron, which is a positively charged electron, and the neutron. So from detection standpoint, that becomes a piece of cake to detect those two particles. And we look at the number of indicators such as the energy of the particles, their timing, and the spatial distribution. So we can correlate those pieces of information to determine, yes, it was neutrino or, no, that was not a neutrino. So that process is well understood and we employ it in practice all the time; it's called inverse beta decay, or IBD for short.
The second question is if the neutrinos don't interact with ever anything, how do you detect them, right? And that has to do with the sheer volume of neutrinos emitted by nuclear reactor. So you can think about it: If the number is large, then the detector can be quite small, right, because the probability of interaction is the same no matter where you put your detector. So if a nuclear reactor emits 10 to the 20, yes, 10 to the 20 antineutrinos per second, now you can factor in the very low interaction probability and counter interact that with a very large detector, right? Avogadro’s number is 10 to the 24, which is what effectively how many protons you get. So now you start talking about a few hundreds of interactions per day, and that is far above any background at neutrinos, the cosmic neutrinos, that you get got it.
Steven McLaughlin: And I was just going to ask—
Anna Erickson: So I had to throw some math in there.
Steven McLaughlin: —and that was perfect. And I was just going to ask is, you know, how are the antineutrinos from the reactor different from the antineutrinos that you say are passing through our body that, I think you also said, come from the Big Bang? But I think you just explained that.
Anna Erickson: Well, there’s a whole ‘nother story to antineutrinos. You know, those particles, people that research them get Nobel Prizes for a reason. And the main reason is they are so elusive and there's so much unknown about that particle which is, by the way, one of the most abundant particles in the universe. The unknown part is that they change their flavor. What that means is they may start at electron antineutrino, but by the time you try to detect them, they may turn into tau antineutrinum. So there are a total of three flavors of those particles. And different detection methods only work for a certain type of antineutrino. So nuclear reactors emit what we call “electron antineutrinos.” That means that when we build IBD detectors, they are blind to any other antineutrinos whether they come from sun or Big Bang. That's the interesting part. So when you know what type of antineutrino you’re detecting, you build the detector tailored for that. And I could go on and on about that because the particle has such a rich history and a lot of interesting aspects.
[marching band music]