Steve W. McLaughlin
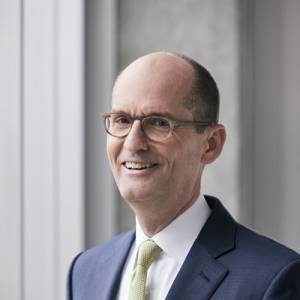

Audio
Audio & Captions
Transcript
[train whistle]
[crowd cheering]
[instrumental Ramblin’ Wreck from Georgia Tech fight song]
Steve McLaughlin: You're listening to The Uncommon Engineer. I'm your host, Steve McLaughlin, dean of the college.
Radio Announcer: We’re just absolutely pleased as punk to have you with us. Please say a few words.
[animal noises]
David Hu: —went to the zoo, and they measured the urination time of all these different animals. It’s a huge range.
Steve McLaughlin: We can learn a lot from the animal kingdom, even us engineers. Welcome to another episode of The Uncommon Engineer. I'm Steve McLaughlin, dean of the Georgia Tech College of Engineering. The Uncommon Engineer is our monthly conversation about the ways Georgia Tech engineers make a difference in our world, in our daily lives, and in ways you might not expect for an engineer. Our guest today is Dr. David Hu from the Woodruff School of Mechanical Engineering. Doctor Hu studies animals, including how they walk, eat, and yes, how they urinate. Welcome to the program, David.
David Hu: Hi Steve. It's great to be on The Uncommon Engineer.
Steve McLaughlin: You know, when a lot of people think about mechanical engineers they think about trains and planes and automobiles and manufacturing and materials, but you study animals. Why is a mechanical engineer studying animals, and how does that apply to mechanical engineering?
David Hu: I'm a mechanical engineer, but I also, in mechanical engineering, I study a field called fluid mechanics, which is the motion of stuff like air and water. Lots of devices based on this, like ships and airplanes. But you know, the very first role models for us mechanical engineers and fluid mechanists were these things like birds and fish. Basically, nature's way to move around in fluids. They've been an inspiration for hundreds of years, but recently there has been technologies like high-speed cameras and 3D printing and 3D scanning that we can actually start to really figure out how these animals are doing these amazing things. And then when we look at these animals, we are hoping that we can use the principles that we see to design new kinds of devices.
Steve McLaughlin: You've gotten a lot of media coverage for some of your work—a splash, can I say—from a study that you did not so long ago about mammal urination. Can you say a little bit more about that, why you got started on that and what it is we can learn from it?
David Hu: Well it all started when I was changing diapers for my son and I had this— This thing happens when you change boys’ diapers. Sometimes they’ll actually just pee on you.
Steve McLaughlin: I’ve been there.
David Hu: And one of the things you noticed when you watch babies pee is that they take an awfully long time. At first, I thought that he had bladder problems, so I watched him. It took about something like 21 seconds. It takes a really long time. And if you go to the bathroom and time yourself, it's about the same. And that's just a very strange— This just aroused curiosity. I mean, I thought that maybe my son had some kind of health problem, because he weighs 10 times less than me, so I should have 10 times as much urine as he does. But he’s—
Steve McLaughlin: His bladder’s got to be one quarter or one 10th the size of your bladder.
David Hu: It turns out it's all proportional, so yeah, a kid’s bladder was a 10th, but it was coming out at the same time and it didn't make any sense. So I recruited some engineers to come try to understand the fluid mechanics of the bladder, and it turns out if you look in the history of this field of urology there's been a huge amount of questions of how the bladder works and how actually fluid comes out. There's controversy over why, if it is gravity driven or you have this special muscle called the bladder, is that pushing it out? And no one really knew. So this is a great example of turning to nature to sort of understand this thing.
So, since humans evolved from all sorts of different animals we actually went to the Atlanta Zoo and we measured the urination time of all these different animals. It's a huge range. Best one to give you an example of is an elephant. So to measure the urination of an elephant, students have to wake up at 5 a.m. when the Elephant just gets up, because elephants don't listen to anything you say. And then they go and put a huge kitchen garbage can underneath this elephant, and the elephant fills this garbage can. It's about 20 liters in about the same time as my son, about twenty-one seconds.
Basically, all these animals have the same thing in common. I figure there's got to be some common principle, because this is not normal. And so it turns out if you look into the anatomy, what the elephant has and what we all have is this special pipe. My kids and I, we call it the pee-pee pipe, but it's called the urethra. And for elephants, it’s a meter long. Little do people know that males and females both have these pee-pee pipes. For female elephants, it’s a meter long and is about the width of my fist.
What the elephant does and what the rest of these animals do to get urine out is that— the wider the pipe, you can imagine, it's like a highway. The more simultaneous lanes cars can go down. So the wider it is, more fluid can come out. But then the second effect is the fact that this pipe is really long, and that uses this Bernoulli effect, where basically if you have got a long pipe you can actually focus the force of the gravity so you can get fluid coming out a lot faster. You can notice this if for example you take—I think some students call it beer keg physics—if you take a beer keg, if you tap a hole in the middle, it will flow out way slower than if you tap a hole at the bottom.
And that's just an example of, you know, where you put this hole makes a huge difference in the speed, and that's basically what the animals have done. They've taken this like really, really thin pipe and infinitesimal amount of fluid and they use that to accentuate the speed so that even if you've got 20 liters of smelly urine, you can get out the same speed as if you only have like a few ounces.
So I started this thing as a curiosity, but it was— it just became— it just became a lot of fun. And it turned out that actually a lot of people, a lot of physicians and engineers, have actually been starting to use our discovery. There's this Japanese doctor who read about our work and he was— So this urologist, he interviewed 2,000 Japanese people all the way up, you know, from kids to adults. And he basically extended our rule—what we call the law of urination, this 21 second rule—and he said he found out that just because of natural aging, basically if you're 80 years old, you're going to urinate at an average time of 30 seconds instead of 20 seconds.
And before this, doctors, when they wanted to measure the health of the bladder, they had to use basic lasers or ultrasound. But now if they want to find out if your bladder muscle is weakening, they can just measure your urination time and show that, oh, your bladder muscles are really weak. I was really happy to see them see this and use our discoveries to sort of help older patients.
There's also the example of— they’re make making prostheses, basically these sort of artificial urethras. And they needed basically a way to test these devices to make sure that they're sort of robust enough to last for a lifetime. And so now they've made it the official protocol for testing urethras is this 21 second rule urination. They'll make sure that it can urinate for 21 seconds every few hours and they'll do this test for days and days and days on these on these products. I mean, I was really happy to see that doing something that was fun could actually influence, you know, scientists from the Netherlands all the way to London and all over the world.
Steve McLaughlin: Let's talk about the frog tongues. Some of your more recent research, again really, really cool stuff. You know, I think as kids we've seen frogs using their tongues to grab stuff. What can we learn from a way a frog uses its tongue for engineering?
David Hu: So this frog tongues project started when I was teaching a class on biology at the Atlanta Botanical Garden. I was just really interested in how they do it. So the first thing we did was we took a high speed camera of a frog. And it's amazingly fast. It can reach out and grab an insect in a thirtieth of a second. It could fit 10 frog strikes in a single eyeblink. And we looked around and we realized that no one really knew why the tongue could grab something so fast. And it seemed like it would have pretty obvious applications, like if you have these micro air vehicles, if they want to actually pick up a cargo, if they want to pick it up before it leaves, they've got to really move fast too.
So this is an example of reverse engineering how the frog tongue works. So I hired this graduate student named Alexis Noel. She became famous for carrying around a bag of bloody tongues. Because one of the very first things that we did was we got tongues from biomedical engineering. One of their dissection classes had these frogs and we got 20 frogs and we opened their mouths. The frog— This is actually the first time I ever touched a frog tongue. The thing I found out it is amazingly soft. It turns out it's 10 times softer than the human tongue. It's like 20 times softer than a marshmallow. In fact, the only material out there that's as soft as the frog tongue is basically the human brain. Nature has basically not come up with anything really softer. That's about as soft as it gets.
If you touch the frog tongue, it kind of feels like a sticky chewing gum, but it has this weird sort of sticky coating. And this coating, if you take it off with your fingers and slide it between your fingers, it feels really weird. It's sort of sticky at first, but then when you slide harder it gets slippery. So this is the property of materials called non-Newtonian fluids. A typical example of this kind of fluid is like paint. So paint was engineered by chemical engineers who wanted a material that— Imagine you put paint in a can. You can stir it with your brush. But then when you put it on the walls, it's got to stick.
So in other words, frog saliva, just like paint, it kind of has like an on-off switch. When you push it really hard, it flows like a liquid, but then when you push it softly it sticks. It just sort of holds its grip. And that's exactly how the frog saliva and tongue work. It's got to be really soft and have this special sort of on-off switch in the fluid. So when it reaches out and grabs the prey, it's able to stick, and then when it pulls it back to its mouth it actually uses these eyeballs that move ultrafast and they push the prey off the tongue. So it's got to be able to sort of stick to the prey and then make sure the prey can come off later.
After we did this frog project, I was just amazed at how little we knew about how well tongues could grab objects. So I thought the next thing to do was to focus on her childhood hobby, which was for her, she just loves cats. She was telling me the story about this cat, Murphy, that it really likes to lick things around the house. And one of the things that it licks is this blanket. The funny thing is when it licks this blanket, it gets its tongue stuck in it and it gets really angry and things like that, but it continues to lick this blanket. And we were talking about this and I realized that's really strange, because the frog tongues are super soft, but these cats, they have actually these spines. I wonder what the heck these things are.
So she went on this adventure around the southeast. She went to— I don't know if you've heard of Tennessee Tiger Haven.
Steve McLaughlin: No.
David Hu: There's a lot of drug dealers around the country and they like to raise tigers as pets, but oftentimes these tigers get to be the size where they'll eat like 50 pounds of meat a day and things like that, and they'll have to give them up for adoption. All the illegal tigers in the U.S., they go to this place called Tennessee Tiger Haven. We went to this place and we picked up the tongues of all these different cats—tigers, cougars, lions—and we found all of them had the same exact spines as her cat. Not only the same shape, the same size, where we actually could start to use some technology.
We actually picked up each little spines from these different animals and we put them in a 3D scanner. And we 3D scanned them, and then we actually 3D printed them. Because the tigers are a little bit too dangerous to really study up close, so what we wanted to do was we wanted to build a device that was kind of as effective. These cats, it turns out they're spending something like 20 percent of their time just grooming themselves. And they've got to do that because they're sort of solitary predators, so they've got to be— even after they've killed a prey and gotten themselves coated in gore and blood, they've got to be able to clean themselves completely in order to go find their next prey.
We 3D printed these little spines and we embedded them in this soft silicon matrix. And so in that way we actually built an artificial cat tongue, and we could actually test how well this thing could work on basically helping to clean fur and different kinds of fabrics. So that was an example where we kind of used the ingenuity in nature, and then we use current technologies to build something that kind of has these properties. And then we can actually help it solve the science as well.
Steve McLaughlin: We talked about tongues, we talked about urination, and I know one of the things that at least I saw was after the flood in Houston, your work on ants and how ants respond to their anthill being inundated with water, or what they do in a flood, and you got a lot of publicity around that. Can you tell us a little bit more about your work with ants and how it relates to floods?
David Hu: Have you ever gotten bitten by a fire ant?
Steve McLaughlin: Oh yeah. Yeah.
David Hu: They're a pretty big deal all over Georgia, and in fact they’re a species that’s invasive to the entire half of the United States. They're spreading all over the world. I was just, a week ago, I was just talking to a radio station in Korea. They were just freaked out seeing fire ants show up at their harbor, and they were worried that they were going to end up kind of like the United States.
And so why would an engineer want to study fire ants? Fire ants are interesting because they can actually survive floods. And that's not typical for an insect. Most insects— There’s these insects called water striders, these insects that sort of— They kind of like, on the surface of lakes, they glide. They're really elegant. They move quickly. Those are the one of the few exceptions. But most insects that stuck in water, they usually just become food for water striders and they drown. But these fire ants, they're one of the few species that actually can survive flash floods.
There are about 10,000 species of ants in the world, and this one evolved in Brazil in this area called the Pantanal. It's a sort of vast wetland that every single year during the rainy season it floods up to a meter deep. Any animal that had to survive this had to evolve ways to deal with water. And so what the fire ant does, it does this amazing thing. If you— And you can actually simulate this. If you have fire ants in your home, you can take a bucket of them, and you just drip water into the bucket. And if you do it slowly enough, you don't drown the ants. The ants will actually come out of their underground homes and what they'll do is link themselves together, and they're going to build this waterproof raft.
By linking their bodies together, they can actually trap air pockets, and that makes the entire thing really buoyant and it makes it really pretty much seaworthy. And as a result, these sort of dinner-plate-sized ant rafts, they can float out to the open ocean, they can last for months just by feeding on their sort of offspring. I know that's kind of gross. They're really, really good at being waterproof.
What we discovered in our lab was that they're not just good at being waterproof, but these rafts, they’re kind of like— they’re an example of— I mentioned this earlier, but is a non-Newtonian fluid. They're an example of a material that's both kind of liquid and solid at the same time. For example, these rafts sort of strike— You can you can take an ant raft and you can squeeze it between your hands and it’ll sort of spring back out, kind of like a Silly Putty. Or you could sort of drop a rock on top of it, and instead of the raft sinking the rock will actually sink. The individual ants will sort of feel the presence of the rock and they'll move out of the way and reconnect on the other side so that the rock actually kind of penetrates the raft like some Terminator material.
It's amazing that the ants can do it because they're living things, and they do it purely by mechanical connections. So they just basically make and break these connections and they can sort of have these properties that make them really resilient. And so this project was actually funded by the Army Research Office. They were really interested in self-healing materials, and I mean ants can sort of give us some kind of idea of how to build a really self-heating material, just like ants can.
Steve McLaughlin: We've talked about the kind of work that you've done in the past and you have shared the work that your students do. Where are you headed? What are the kinds of— What's next for you and your group?
David Hu: It's sort of top secret, but since we're on this Uncommon Engineer show, I guess I have to tell you a little bit about it. This is not published yet, but my students actually, we entered a competition last year, an international competition in Montreal, to build a sniffing machine. To build this device that can distinguish different types of cheese in Montreal. And so that's actually pretty hard. I mean, a human can do it, but how do you build a machine that can distinguish not just, you know, alcohol and water, but different types of cheese from northern Montreal and southern Montreal—brie, camembert.
We built this device that uses these principles of animals. In biology, this has been a longstanding question of why in the world do animals sniff? Like, when I have dirty gym socks, I don't take a long whiff. I'll do these sniffs. And if you look at your dog, they'll do the same thing. And it turns out it's this principle that's been observed. We've done experiments at the Atlanta Zoo. Elephants also sniff. Why in the world are these animals sniffing? Why does taking short periodic breaths, why does that help you distinguish objects better? So we built this device that resembles a bellows. We call it Gromit. Gromit is this character that went to the moon to figure out if it was made out of cheese.
Gromit: Fancy cheese! We’ll go somewhere where there’s cheese!
David Hu: It basically has a bellows that pulls and pushes air around a sensor, and we found out if we basically emulate the animals sniffing rates—for a dog that's about two to almost eight times per second—we could actually get the sensor to read much more data from the cheese than without. And so, with this device and this sniffing mechanism, we were able to actually get third place in this international sniffing competition.
That meant we got to eat all the cheese. But I'm really happy about that because I think there's a huge amount of fluid mechanics outside swimming and flying, too, like our senses. Sense of smell really requires on fluid, air, as a carrier. And it's really— You can probably imagine it's also like a programming problem, like how does your brain distinguish these things. How do you bring those messages to your brain in the most effective way?
And so we're building these devices that kind of look like an elephant's trunk. Elephants apparently have a really good sense of smell. They’ve got more nasal sensors, more neurons for smell than any other animal, and no one really knows why. So I really want to understand fluid mechanics of different senses. So I went from basically the tongue and now I'm going to the nose.
Steve McLaughlin: One of the things that we always do on The Uncommon Engineer is we talk about what got you to thinking about being an engineer. Can you say a little bit about where you grew up and some of your experiences in childhood and how that drew you to becoming an engineer?
David Hu: I've always loved animals. I think what I liked about animals is they're really complicated. I didn't really have a way to study them until I went to college. Actually, before college, I didn't even know what engineering was, but I took this class in mechanical engineering because I really liked my physics classes. I liked the physics of the everyday world. That's the first physics class people take, this thing called mechanics, where you learn like about how bridges work and doorstops and things like that. And I thought that was interesting, and I wanted to learn more about materials and things like that, about things that you can touch.
And so I started studying mechanical engineering, and I had this undergraduate adviser and I remember that in his office he had this cover of Nature magazine. It was about intercellular springs. And he had basically come up with models for how cells work, and how they actually used— And I had been studying springs in my engineering class, and I said, wow, that's something that doesn't look at all like a spring but it sounds like those principles are being used to understand like really, really different things. And so then I started getting interested in— I thought, hey, I think mechanical engineering would be the right tools that I would want to use. And now I can actually start applying them to my childhood hobby of looking at animals.
Steve McLaughlin: And now you're a professor, you work with students and you teach, and so you could have gone off in a different way as a mechanical engineer. And so what drew you to wanting to be in the classroom or do research or work with students?
David Hu: So I got my Ph.D. actually in mathematics. My grants are in physics and I'm a joint professor of biology. So I've had sort of lots of different sort of invitations to go in different directions. I really think mechanical engineering is still the best place for my kind of work. I mean, all of the students that I've worked with in all the projects—these cat tongues, this model of urine and this cheese sniffing device—those are actually— they're all built by engineers. And that's the thing about engineering, I think it's sort of a very, very powerful tool. And all I've done is sort of brought a different place for them to apply it.
The biologist gives us a huge number of questions that we can answer, and we're using a lot of the tools that are available made by Georgia Tech and in my department. We've got this thing called the Invention Studio where we can— that's where we 3D printed and 3D scanned these cat spikes and these cat tongues. There’s these verometer devices that are used by chemical engineers. So basically, we have all the tools here and I just sort of bring the questions, and I think the two of them together and this is whole engineering mindset I think is really how it works. Like the students I have, they want to figure out how things work. They're not satisfied until they know exactly how it works and understand how well it works so much that you can actually build something that can do that process, maybe even better or faster.
Steve McLaughlin: I think our listeners can already get a sense of what makes you an uncommon engineer, but in your own words, what makes you an uncommon engineer?
David Hu: I'm an uncommon engineer because I see engineering in the everyday world. I look at my cat, my dog. And I look at these animals and I see all sorts of engineering principles and I see how amazing they are and I want to use them to build better devices.
Steve McLaughlin: We're really lucky to have you at the Georgia Tech community. Keep doing what you're doing because I think it's really, really important for young people to really understand that love of animals can turn into a really, really fascinating career. Thanks so much for coming today David.
David Hu: Thanks a lot Steve. It's been a pleasure.
Steve McLaughlin: For now, that's all for The Uncommon Engineer. Be sure to tune in next month when we'll talk to Dr. Jonathan Rogers on how drones are shaping our lives. I'm Steve McLaughlin. Thanks for listening.
[instrumental Ramblin’ Wreck from Georgia Tech fight song]
Geekout
Audio & Captions
Transcript
[analog radio tuner scanning stations]
[big band swing rendition of Ramblin' Wreck from Georgia Tech]
[interposed voices of Steve McLaughlin] ...sounds incredibly complex...it sounds like...to have abilities that span...I'm really geeking out here.
[applause and laugh track]
[big band swing rendition of Ramblin' Wreck from Georgia Tech]
Steve McLaughlin: You really have this incredible ability to explain complex ideas whether they are biology, whether they’re engineering, how they apply to practice. And that's, you know, one of the things that engineers are often criticized for is we don't do a very good job of communicating to the general public what we do, why we do it, and so on. And I know that that's a passion of yours, you know, in addition to the science is to try to, you know, have engineers, scientists do a much better job of communicating what it is they do, because it's incredibly important and exciting to attract young people to science and engineering. Can you say a little bit about how your interest in engineering evolved, and then how you're so passionate about making sure the public knows what engineers do?
David Hu: I do really like telling the story behind the work. We have the journal articles where we’ll publish the technical aspects of the work. But a lot of times, you know, what I remember is the same kind of story that I'm going to tell my kids and I'll tell my grandkids of, you know, who are the people behind this story and what were the challenges and why was it difficult. And I just really enjoy that process. And one of the reasons that I continue doing it is because it's has actually helped my research quite a bit as well, and that's something a lot of, maybe, scientists and engineers don't know. For example, when we first started studying this urination, we published this article in National Geographic. And this lawyer from New York City sent us an email and it gave us a correction in the article. He said that he used to work in zoos when he was a kid and he said, “Well, elephants actually have a slightly smaller bladder for their body size than according to these evolutionary trends. So you should make this correction in your paper.”
There is no way I could have gotten in touch with this guy unless I had put this out in National Geographic. So he actually influenced our paper, so that we actually when we published the final article in Proceedings of National Academy of Sciences that it was correct. So, and that's just one example of when you reach out to the public, there's a huge, huge number of people out there that are kind of these like citizen scientists that they give feedback, and they just—it's like having a million eyes. They kind of like help you at once. And, I mean, it's just, I think it's a lot of—it's also just fun, it's fun to get to know them and they know there's this huge, huge amount—especially about biology and about nature that the general public knows about. And I really try to use them as experts, too.
Steve McLaughlin: As you were talking about the properties of this kind of ant raft, I was trying to envision the tools that you use to really get this incredibly detailed information. You said you use high-speed cameras. Can you say a little bit, you know, from a technical standpoint what are the kind of tools of the trade for someone who does what you do?
David Hu: The nice thing about being an engineer or scientist, we get to play with really cool devices. The thing that you want to use to study ants is this thing called a rheometer. Now it was actually, this rheometer, it looks like a little merry-go-round except it costs like $60,000. That’s like more than the price of my car, way more. And the rheometer was invented by chemical engineers who wanted to basically engineer food products. For example, M&M’s, they melt in your mouth but not your hand. Yogurt, has this what they call “mouthfeel.” All these products have been engineered by adding different additives to make them taste a different way. And what this rheometer does it has these two spinning plates, and what it does is it can actually measure the properties, the materials, really precisely. And so we are the first ones to actually put sort of living organisms in this rheometer, but we basically treated them as this, you know, alien substance that we kind of wanted to reverse engineer.
Steve McLaughlin: I see. So just, again, using regular tools of the trade that, you know, that engineers use. Like you said, you take take the animals or, in this case, insects, as just another, as another thing that you want to test. So it's totally normal from an engineering standpoint.
David Wu: Yeah. In our lab we borrow the rheometer there. In their lab they have these two rheometers. One of them they call the “clean rheometer,” and my realm is the “dirty rheometer” because we'll put feces and ants in it, and we have to have this vacuum cleaner around in case the ants escape. So yeah it's a—you basically—we make special engineering tools a little bit grosser.
There's this animal that we're studying called the star-nosed mole. No one's ever heard of it, but it's just a mole that can actually sniff underwater with like basically our nose. So if you go scuba diving, you can’t actually smell the underwater. All you smell is that sort of disgusting rubbery mask that you put on. And that's because there's an air water barrier and we can't penetrate that. But a few of these small, sort of small, rodents like the mole, they've actually evolved a way to blow bubbles underwater and inhale those bubbles back in to its own nose. And so it's basically sort of hacked, sort of this system, and made its ability to basically smell under water. And it uses this to sort of catch worms. It's almost completely blind.
Steve McLaughlin: That's what I was gonna say. Why does this mole need to smell underwater? And you're saying it uniquely adapted because it's a better worm hunter than maybe others.
David Wu: Yeah, that's right. It basically has to completely rely on the sense of smell to go in an environment where its nose wouldn't normally work. And so it exhales and inhales these bubbles. And we think we get basically—we're using our grommet, our device that we use on air, and we want to put underwater. And the great thing about this is that we would actually be able to give a whole new regime to sort of above-water sensors because right now there's no solution for sensors underwater.
I was at Auburn a few weeks ago and looked at their aquaculture tanks. The raising of fish is a huge, huge rising industry. But there is no permanent way to actually sense things underwater because of algae, bacteria. The closest device they have is this brush that periodically goes over your sensor underwater, but eventually the brush gets clogged. So we can basically take advantage of this, you know, this star-nosed-mole and we can build this sensor that basically never actually has to touch water, but can actually smell underwater. I think, I think that would be a pretty big deal.
Steve McLaughlin: Now, I mean, again, as you were talking, I’m thinking, why in the world do we need to you know smell things underwater? But that's probably because we've never been able to do it. And if, in fact, we're able to develop the systems or the sensors that might develop from that, it's really, really quite something.
[tribal percussion, birds chirping, monkeys chattering, elephant trumpeting]
[Ramblin' Wreck from Georgia Tech marching band rendition]