Steve W. McLaughlin
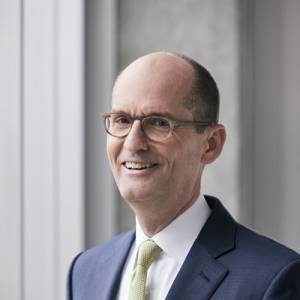

Audio
Audio & Captions
Transcript
[steam whistle]
[marching band music]
>> I'm Steve McLaughlin, dean of the Georgia Tech College of Engineering, and this is The Uncommon Engineer.
[music continues]
[archival recording] Announcer: We're just absolutely pleased as punch to have you with us. Please say a few words.
[applause]
[big band music]
Steve McLaughlin: In many developing nations across the world, drinking water and sewage system infrastructures are nonexistent. This leads to unsafe sanitation issues causing public health concerns. To fix this, the Bill and Melinda Gates Foundation challenged top scientists and engineers to build a better toilet that doesn't require running water.
Welcome to another episode of The Uncommon Engineer podcast. I'm Steve McLaughlin, dean of the Georgia Tech College of Engineering. The Uncommon Engineer discusses how Georgia Tech engineers make a difference in our world, in our daily lives, and in ways you might not expect.
[music]
Our guest today is Dr. Shannon Yee. He's a professor in the Woodruff School of Mechanical Engineering here at Georgia Tech. His research is focused around thermodynamics and energy transfer. He's taking that knowledge and trying to develop a toilet that uses heat transfer to break down wastes instead of water. Welcome to the program, Shannon.
Shannon Yee: Thank you. It’s great to be here.
Steve: Yeah, well, we're really happy to have you. You know, today we're going to talk about toilets. You know, a lot of people—it's a topic a lot of people don't want to talk about. A lot of people are aren't necessarily familiar with. You know, it’s something we take for granted in the U.S. and in Europe, but why are foundations like the Gates Foundation and yourself working on toilets?
Shannon: I think the short answer is there is a lot of individuals out there who do not have access to improved sanitation. If you break it down, there's about 2.5 billion people around the planet who don't get to enjoy the luxuries that we have when it comes to sanitation. It's also a major global disease vector. I don't think people realize that about 80 percent of feces end up in water, which is why we spend so much energy and resources cleaning up water so we can drink it. If you can keep feces out of water to begin with, then we have an easier job later.
It really is a problem for everybody is the short answer. The developing world is of particular interest because of pathogenic vectors. But even for the developed world, our sanitation systems are about a hundred years old, and they're aging pretty rapidly right now. When we look at it, probably are looking to do some massive replacements of that infrastructure over the next 30 to 50 years. What I worry about is I don't think people realize how expensive those infrastructure improvements are going to be. And in some cases, even in the developed world, we may not be able to afford that.
Steve: So yeah, and so before we get into some details that kind of the image that just brought to mind is, you know, even in the most developed countries, you know, something that—so in the less developed world, I can imagine toilets that are kind of self-contained, don't require plumbing, or don't require sophisticated plumbing like we have, and I think you're saying, you know, kind of more modular or single-unit kinds of things, and you're saying maybe even in the developed world, because of all this decaying infrastructure, that kind of model might work for us, too; we don't necessarily need to be tied to the infrastructure we have, and it may be a big advantage. And I know that you've received a large award from the Gates Foundation, the Bill and Melinda Gates Foundation, which I think a lot of people are familiar with. So maybe talk kind of what are the specific challenges that the Gates Foundation is interested in and which are the challenges that you're addressing in your lab.
Shannon: So the overall challenge for the Gates Foundation project is to deliver a sanitation system that is very low-cost. And the cost that we're looking at is about five cents per user per day; that’s kind of a generic cost. When I break that down to an individual toilet, it comes out to a capex cost about $450 for the unit with an operational expense cost about 15 cents per day. So those are the cost targets we're looking at. And when you translate that, that becomes affordable then to the developing world. It comes in at about three and 3 3/4 cents per user per day with those targets. That's one of the major drivers.
The major technical specifications we have to hit are really holistically trying to limit oral-fecal pathways. So how do we actually prevent oral-fecal contamination?
Steve: So, I know it’s a little uncomfortable to talk about, but feces that ends up in the water that ends up being consumed.
Shannon Yee: That's exactly right. In our current infrastructure, we rely on mixing feces with water. We have very, you know, we have flush toilets, right? And then everything goes down a pipe and you forget about it. And so we use water primarily as a transport mechanism to the centralized facilities. However, when you look globally where population growth is currently predicted, a lot of the fecal sludge management, about 40—sorry—74 percent of the fecal sludge management zones are actually in areas where water is a scarce resource. So how do you start treating waste without water? And that's why there's a need to move away from a centralized system and look at how you can do it in a distributed system.
Steve McLaughlin: Well, this this certainly can't be a new problem, I'm sure. Society, we've known this for a long time, decades if not hundreds of years. And so I'm really curious about what the challenges are, you know, kind of getting to this point, and with the Gates’ challenge that you're involved in, what it's addressing.
Shannon: Yeah, most certainly. So, you're right—it's not a new problem. People have been having to deal with their waste for quite a long time. What we're have been facing over the last, let's say, a hundred years, maybe a little more, is we see a kind of a dense urbanization; we have people moving towards cities. And so with that, we had kind of the advent of the flush toilet and the S-trap and the P-trap, which has really modernized a lot of our sewage infrastructure. And that occurred right around 1900s. So it's been about a hundred years now since we've seen kind of a re-imagination of that system. And so about a decade ago the Gates Foundation realized this and said, “You know what? We need to help nucleate a change in how we look at sanitation.” And so up until that point, I would say the field was really kind of stagnating; there weren't really new inventions to come that were developed.
So back in 2010, 2011, the Reinvent the Toilet Challenge was launched in 2011. And with that a whole bunch of grantees came to try to work on this problem. And over the last nine years, those grantees have been nurtured and there's now more of a scientific community. There are a couple of new ideas and patents, and there's some different prototypes that have been developed around the world to address this problem. And what we're looking at here, and the question I posed to the Gates Foundation, “Have we done enough science to really deploy engineering at scale?” And I think this is where Georgia Tech comes in as a unique partner with this because we can really start bringing together many different disciplines. And with our strengths with the Georgia Tech Research Institute, we can really pull that team together. Where we are now is we're trying to be hyper collaborative, and it's not a competition; we're not trying to compete against each other to see us the best idea. We're now trying to look holistically across the entire portfolio and go “What ideas work best,” because not all the best ideas originated at one group. There are really great ideas with multiple different groups and how can we pull the best ideas and then collaboratively come together with a really ideal solution.
Steve: I'm ready to hear about some of the interesting ideas and solutions or the ones that you're working on or the ones that you're most excited about or where you think it's headed.
Shannon: Definitely. We're still in the early phases of this. We kicked everything off in May, and what we're doing in this first 12-month period is we're having a series of design workshops where we're evaluating the different modules, so the different concepts that come through.
The first design workshop we had was to look at the overall system. All right, what choices do we want to make about how this system is going to work because that will influence the individual modules. At the end of this month, we're gathering everybody in Switzerland and we're taking a look at specifically the fecal processing module. And there are numerous ideas for how we can actually do the fecal processing. There are largely—or at least I largely categorize them into three buckets. One, we refer to as “wet oxidation.” One I refer to as “gaseous oxidation,” and one that I referred to as “rapid oxidation.” For the case of gaseous oxidation, that's kind of like drying everything and then combusting the feces. So that's been the dominant choice for a lot of the portfolio to date. And so we want to take a look at that and see, OK, is it on the right cost trajectory? ?an we manage a stable flame or a smoldering front at such a small scale? What we've learned to this point is that combustion works, but it works at a slightly larger scale than the individual toilet. And so we're still trying to evaluate are there unique engineering solutions that allow us to get it down to that individual toilet scale.
When it comes to what oxidation, I kind of refer to this as we're trying to burn under water. Well, we're not actually burning anything under water; we're going through an oxidative process in the presence of water. And there've been other groups that have looked at this; there's been a couple of universities, couple of national labs. And that process is largely categorized into kind of three areas that we're looking at. One is subcritical wet oxidation. Another one is supercritical wet oxidation where we operate at temperatures and pressures higher than the critical point for water. And the third one is what's known as hydrothermal carbonation where we actually increase the temperature and pressure to a point where we start getting polymerization is the short answer. We actually get a carbonization. So the mass just starts forming long polymer-like chains. And so we're evaluating different modules right now and different processes that will go into this overall system.
I think what makes this process different than any of the previous attempts is we really are focused on separating the streams upfront. So we have different modules for processing urine than we have for processing feces than we have for processing flush water. So we look at processing those in different ways. They have different energy demands on them. And by processing three separate streams, we think we can get to a lower-cost, lower-energy system.
For this problem, right, if we want to achieve low cost, we really have to achieve a highly energy-efficient system. So focusing on heat transfer and thermodynamics really allows us to be on a trajectory to achieve a low-cost system. And it's just really making decision choices based upon thermodynamics and heat transfer that really, I think, make this a unique system and a unique approach.
Steve: I assume there's a cultural element that you must be thinking about for toilets. So I know we're off topic, but I'm really curious about that.
Shannon: So there's a very prolific YouTube video where Bill Gates was drinking water out of one of these processes, right. There are ways that you can take it to potable levels. The best way I can think of is if you combust everything, one of the combustion products is water vapor, and then you can condense that water vapor. It's kind of like a fancy distillation process. So it's possible, but I really think it depends on the business case and the cultural acceptance for that specific region because the solution, when we think of a toilet, it's not just one solution. One thing I've really—and maybe I shouldn’t admit to this—but one thing I've enjoyed is now when I travel, I look at the plumbing fixtures around the world, even around the country. They're very different. There's all these different styles and different ways of connecting up, and there's unique cultural aspects to every single bathroom. And so taking that lesson just from global observations, we're going to have to come up with multiple prototypes, not just one. And that's, I think, something that's unique. We're targeting six culturally acceptable use cases. And why six, right? When we look at it, we have people around the world who practice wiping and people who practice washing. So wiping is using toilet paper; washing is using anal rinse water. We have people around the world who use pedestal toilets, and we have people who use squat plates. And we have people around the world who need flush water. They associate flush water with cleanliness, so we need to include flush water. And there are places around the world where flush water is a luxury, and so you may want to use a dry flush alternative, so not using flush water.
So it gives us a two-by-two-by-two matrix, or eight different use cases. However, when we look back at it, we don't see a use case for washers who want a dry toilet because if you're washing, you have water, so you don't want a dry toilet. So that removes two use cases. And so when we look at this, we see six culturally acceptable use cases. And so we're looking at building six different prototypes.
Now a lot of those use cases are on the front end. It's how they interface with the user and what we can do is we can actually keep a lot of the similar back end processing. We may have to tweak things based upon separation efficiency or, you know, more toilet paper or less toilet paper, that kind of thing. But the core processing technology can be the same behind the wall, but it's how the users interact and interface with that. You know, why we're focused on the toilet, if we're really interested in obloviating oral-fecal pathways, one of the other things we need to consider is handwashing, and it's one of the most effective ways of doing that. One of the challenges here though is soap is a really difficult product to use. So if I have to treat soap locally, those surfactants really clog up some of the membranes, and it becomes a challenge. When I look at handwashing without soap, the CDC recommends using hand sanitizer. So the CDC recommends using hand sanitizer and with hand sanitizer, a lot of it is alcohol-based. There's been some really good studies looking at, OK, how do we distribute hand sanitizer around the world? And I guess use cases in Kenya and Bangladesh, the costs are about three $3.70 cents per liter. And when I look at that cost, that would consume about 75 percent of my opex budget just on hand sanitizer. And so when I look at this problem, like wow, we can't even afford appropriate hand sanitizer, so we need to think of really clever ways at which we can design a handwashing station that can operate and reuse some of that water at very low cost.
There's other challenges in the bathroom that we have to be aware of. We really have to think holistically. One thing we're looking at treating are feminine hygiene products. So how do we handle those and dispose and sanitize them in an appropriate manner? And then how do we handle family products—diapers, condoms, those kind of things? And so looking at all of that, we have to figure out how are we going to address the larger problem, and it's not just the toilet. So those additional auxiliary technologies are also things that we're looking to design and build as well.
Steve: Well I know some of our listeners will be really curious about the other kinds of cool stuff that's going on in your lab. What kind of stuff do you have happening?
Shannon: Yeah. So as I mentioned earlier, my lab focuses on energy conversion, so primarily thermal energy conversion, so how do we convert heat to another form of energy like electricity. And the common example that people kind of point to is this concept of thermoelectrics. So thermoelectrics are devices that can take heat and generate electricity without any moving parts. And they can also take electricity and generate cooling. So one thrust for my lab has been developing plastic, or polymer, thermoelectrics. Where the same thing as you could do with, I guess, rigid semiconductors, we can now do with low-cost flexible plastics. I think this is a really exciting area because we've demonstrated a couple of devices. We have one in the Georgia Tech logo that's printed onto a shirt. And so your shirt using these thermoelectric polymers can convert the heat from your body directly into electricity, which I think is really cool. What I'm more excited about is actually the reverse operation: How do we apply electricity and then deliver personal cooling?
So we've done a number of undergraduate student projects with Capstone developing personal thermostats with a company called Embr Labs. Essentially it's a little thermoelectric device that's connected to a battery that you would wear on your body. Their product on the market right now is a little device that goes on your wrist, and what it does is it sends a cold pulse, a cooling sensation, to your body to make you feel cooler. So right now they're doing it in a little watch-like architecture. We've helped work with them in developing neck-like architectures. But I'm really excited to see how we could then integrate that more into textiles. So textile integrated thermoelectrics, and then I can give you a shirt—you clip a battery onto that shirt, hit a button, and it can make you feel more comfortable. It can heat you; it can cool you. It can really address the personal comfort challenge.
And people look at think, “Oh, this is nifty and neat,” but the core problem that it's trying to solve is one I referred to as “global cooling.” This isn't cooling the planet; this is providing cooling technologies for the world. We just saw a couple of weeks ago heat waves in the U.S., right, and we saw sustained heat waves in Europe, and we saw in northern India really sustained heat waves. When I look to the future we need to be able to deliver low-cost cooling technologies globally, so that's one area my lab focuses on. We develop thermoelectrics for that purpose. We look at electrochemical coolers, how we can do that, magnetocalorics, electrocalorics, thermoacoustics. There's all these different conversion mechanisms that we can use to produce cooling. And it's, I think, a need we have for the future.
Steve: Super, super cool stuff. I'm really curious about how you found your way to being an engineer, whether was as a kid going through elementary, junior high, high school heading into college, how do you decide—what was your path to being an engineer?
Shannon: I think I was very lucky and I would say it found me more than I found it. I always, when I was younger, I always tinkered, right. I would always build little things or try to modify little things and I took a lot of things apart and most of the time I could put them back together. And then when I went off to college, I was determined to be a math major because I was really good in math right. And then the more I thought about it, the more I actually enjoyed applying that knowledge and not just working through it. And so I'm like, OK, what allows me to be very broad, right? And that's how I kind of stumbled across mechanical engineering. When I was going through my courses, it was then the topics associated with energy and heat transfer that really inspired me. And then, like many people, I think I had some really fantastic professors who helped nurture that interest, and they kind of guided me along this trajectory. And then with that trajectory, I got to work on some really large energy projects around the country, and energy is really where I'm focused on.
Steve: One of the questions that we ask all of our guests is what makes you an uncommon engineer?
Shannon: And you're going to first have to tell me what a “common engineer” is
because when I look at engineers, you know, one trait that I see is they're problem solvers; they go and attack difficult problems. So I don't know if I'm any more uncommon than any other engineer. I like going after and addressing problems. I think the problems that got me most excited are the problems that have large global impact. So I want to look at problems that can affect billions of people's lives or they solve one of the grand challenges that our world is facing. So I get really excited about trying to come up with a contribution or a solution to a really, really large problem. I know a lot of people find that to be very daunting because the problem is so big. I don't think I'm going to solve all of it, but I like trying to figure out what small contribution I can make to a large problem. And that's one of the things I really instill in my students. It's like, “OK, you're working on something. Tell me about the motivation. What problem are you solving? What are we actually doing?” I think the most precious thing we have is time. And I want to use my time to solve big problems.
Steve: I appreciate you being on the show. And most of all, I know I speak for myself and a lot of folks, we're really lucky to have you here at Georgia Tech and the influence you have on our students and the difference that you're making. And so thanks so much for being here and see you around.
Shannon: My pleasure. Thanks for the invitation.
[big band music]
[marching band music]
Geekout
Audio & Captions
Transcript
[scanning radio station dial]
[big band swing music]
Steven McLaughlin: —sounds incredibly complex—it sounds like—you need to have abilities that span—I’m really geekin’ out here.
[laughter]
[big band swing music]
Steven McLaughlin: Probably most of our college students know a little bit about thermodynamics, but high school students and others—could you just say a little bit what you mean by thermodynamics? And you just described a whole bunch of different places where thermodynamics comes in, and so how does it relate to toilets or mixing or the project that you're working on?
Shannon Yee: Definitely. So what my lab focuses on, right, is looking at the interconversion of heat to other sources of energy. The tagline is we focus on direct thermal energy conversion technologies and thermal transport technologies. And what does that exactly mean, right? Fundamentally, I think people are aware that we have flows of energy in the world around us. One of the most common flows of energy is heat. We kind of classify heat by the common modes of heat transfer that we encounter. We have conduction, which is the transfer of heat and solids. We have convection, which is the transfer of heat between a solid and a fluid or between fluids. And we have radiation, the transfer of heat through light. And that kind of forms a fundamental scientific base which we can then apply to different technologies. And what I'm always interested in is how can we convert heat to another form of energy. And that conversion is largely described as thermodynamics: how do we take heat, thermal energy, and convert it to something else.
And so that's kind of the underlying thrust for my lab is how do we better utilize heat. Sometimes we use it to generate electricity. Sometimes we use it to generate mechanical motion. Sometimes we use it to generate chemical species. We can also run all those in reverse. So I can use electricity and I can provide cooling technologies like air conditioning. I can take even nuclear energy, beta particles, and I can convert that to electricity. So it's just really the interconversion of all of these different energy forms that kind of encompasses what my lab does. Because heat is such a large component of our energy sources, we focus a lot on how can we transfer and move heat and use it most effectively. And then the utility of heat in a very efficient manner, that's also an underlying principle for thermodynamics.
The approaches we're taking once we have these separated streams in a toilet is our goal. Our specification is to really obliviate fecal-oral pathways. And the challenge within feces is bacteria and viruses. So when I look at that, my job is to really arrest bacteria and viruses. And so one way of doing that is heating everything up. So if we can elevate the temperature of that waste stream for a sufficient duration, that largely ends up killing most of the pathogens. So that's one of the first approaches that we want to do within this toilet. The problem there is, even if I kill everything, I still have a very nutrient-rich stream and there's enough naturally occurring bacteria in the air that if I just discharge that nutrient-rich stream, a lot of these bacteria will come back. So we're concerned about regrowth.
Steven: Even just regular ol’ contact with any other environment that has bacteria in it?
Shannon: That's right. So if I, you know, if I have this pasteurized waste and I just leave it exposed to air, things will start growing again. And so that doesn't solve the ultimate goal of a obliviating fecal-oral pathways, so we have to take it one step further. And what we have to do is take that sanitized waste and we have to make it devoid of energy. We have to remove all of that energy, and that's why we'll look at other chemical and physical means to do that. So once we, for example, let's say we were going to combust it. Once we take it to a form of ash, things won't spontaneously regrow because we've removed all the energy from the system. So we have to look at how we remove that, but what do we do with it? It'd be a shame just to waste it, right? And so a lot of the concepts are how can we take that energy and actually utilize it.
[archival recording] Narrator: Cold is only the absence of heat. And heat doesn't just disappear; it has to be carried away.
[marching band music]