Steve W. McLaughlin
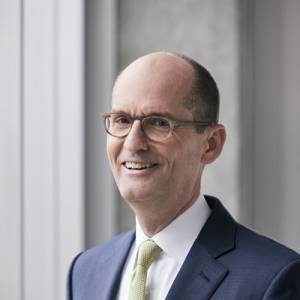

Audio
Audio & Captions
Transcript
[steam whistle]
[“Ramblin' Wreck from Georgia Tech”]
Narrator: I'm Steve McLaughlin, dean of the Georgia Tech College of Engineering, and this is The Uncommon Engineer.
Archive: We're just absolutely pleased as punch to have you with us. Please say a few words.
[applause]
[distorted music]
Archive: The enzyme-containing solutions are collected in special tubes, which are placed in centrifuges. Spinning the tubes at 17,000 revolutions per minute separates solid particles of tissue from the enzyme in the solution. The tissue particles have been forced to the bottom of the tube, while the enzyme stays in solution.
Archive: One might assume that these methods provide us with a relatively pure enzyme. However, much remains to be done to get the enzyme… New ideas for accomplishing this task are still very much needed.
Steve McLaughlin: Without insurance, our medical bills could be thousands of dollars with each routine trip to the doctor, and some of the care we need isn't covered by insurance at all, like hearing aids, for example, which can run you close to, say, $5,000.
But why do they cost so much?
[“Ramblin' Wreck from Georgia Tech”]
Steve McLaughlin: Welcome to another episode of The Uncommon Engineer podcast. I'm Steve McLaughlin, dean of the Georgia Tech College of Engineering. The Uncommon Engineer discusses how Georgia Tech engineers make a difference in our world, in our daily lives, and in ways you might not expect.
Our guest today is Dr. Saad Bhamla. He's a professor in the School of Chemical and Biomolecular Engineering here at Georgia Tech. He's gonna talk to us about his work and what people are now calling “frugal science” and the impact it's having on the medical field. In fact, his new hearing aid is only going to cost $2. Welcome to the program, Saad.
Dr. Bhamla: Thank you.
Steve McLaughlin: So let's start with you explaining just what the idea behind frugal science is. I think people understand the word “frugal” and the word “science,” but it's a research area now. So can you explain what it is?
Dr. Bhamla: A part of my lab focuses on this philosophy or research theme of frugal science. The way we think about this is that we share this planet with about 7 billion other people. We don't have infinite resources. And there are these grand challenges that we are faced with, whether with human health or even planetary health. And so the motivation for us is, how do we think about solutions and develop technologies that scale, not just for you and me sitting here in Atlanta but for billions? And so our philosophy is can we think about it at—you know, we choose a number and it has some meaning and I can explain is a dollar, which you know, for where I grew up from in India, an annual health care budget for an average person is about $1, $2, $2.50. So often our devices, which are focused on enabling science or health care. If he asked somebody to buy or sell something which is hundreds of dollars, that's just ridiculous. So we choose a dollar, because that's kind of the box we put us in. And that's the frugal. Most of our devices typically cost about a dollar. And then we kind of think outside the box of this one dollar to come up with solutions.
Often with our inventions, it's not just a matter of engineering. Often we're pushing the limits of our own ability to choose new materials that might not have been used. And although we're almost, in a sense, reinventing things… say, think about reinventing the wheel if today you and I were to reinvent the wheel. Would we use the same materials that we had access to, say, about 100 years ago? Would we think about access to new materials? And so it's essentially allowing us to use what we know today as scientists about the physics of materials, soft materials, actuators and ask what is possible within the constraints of keeping this at a lower cost?
It forces us to think a little bit more scientifically, and often with these devices it's not a matter of solving a problem but building key workhorse devices or platforms as we were discussing earlier. So it enables other people. Often we have to think a little bit more scientifically on what are kind of the limits on this thing and what's possible.
Steve McLaughlin: And I think with what we're all used to, I think, as scientists and engineers, mostly as researchers, when we think big, we think about solving big problems. We think about oh, that's expensive, right? To come up with a solution for a big problem. And I will—I'm guilty of that, thinking that those big solutions are routed or rooted in something big and expensive. And you're saying what if we just start from the beginning and totally turn that on its head and say let's start with cost and then ask what problems can we solve? And like you said, arrive, take a completely different path to a solution by starting at that point.
Dr. Bhamla: What I have in my hands is essentially a piece of paper. It's a circular piece of paper that I have A fishing line, a string just poured through two holes in the center of this disk and the strings are attached to PVC pipes that you might have in your kitchen or your garage. And I'm gonna spin it and I'll let you kind of, you know, use your sense of hearing to see what it sounds like.
[whir]
Dr. Bhamla: This object is what we call a Paperfuge. And this is our take on a low-cost centrifuge. So this is one of our frugal science devices. So I have a question for you, Steve. When I spin this—and you can hear the sound—how fast do you think this object is rotating?
[whir]
Steve: It's whirring pretty, pretty loud, so I'll think in terms of revolutions per… revolutions per second. How many times is this spinning each second? And I'm gonna say, I don't know, four or five, something like that?
Dr. Bhamla: It's actually 25,000 RPM.
Steve McLaughlin: That's a heck of a lot faster than four or five revolutions per second. Incredible. That's incredible.
Dr. Bhamla: You know, the problem that we were trying to solve is centrifuges and we had just come back from the field in Uganda. What we were trying to do, diagnostics for malaria and for helminth. And the context of this problem is that even if you and I were to go to, say, Emory Hospital today, a common test that people do is take your bodily fluids, whether it's urine, stool, blood, and often you're looking for some biomarkers or infectious diseases. You're looking for parasites. So the challenge always is finding needles in a haystack. And you have to concentrate things and that's what a centrifuge is. It's kind of a workhorse for any biology lab, whether you think cell biology or biomedical lab.
And here we were in the middle of this place where there was no electricity, so you have a centrifuge. It's not as if you don't have one. But it's akin to, you know, just a big piece of metal just sitting there useless.
So we'd come back and, you know, realize that without this we can do diagnostics and we have a lot of patient data that just you can’t do. And so we were challenged with trying to find a clever way of doing this. And as all the scientists and engineers do, we look in our kitchens and our garages. And we discovered that other people had come up with ideas like egg beaters would be one, salad spinners would be one. And those devices go about a few thousand RPM. It turns out that that's not enough to actually separate out plasma from red blood cells to do a simple test.
So we were looking for what is the upper limit? And so this is where the science comes in. The way I thought about it, from my physics background, is you know what is the most efficient way to actuate an object such that I can apply my muscle energy, my human hand energy, to rotate an object?
And so the a-ha moment came because my grandmother used to give me these buttons to play with as a kid. And so I don't know if you've played with this as a kid, but this is where the inspiration was. And when I put this on a high-speed camera to visualize this because it made this noise, it turns out it's not a few hundred RPM. This spins at 25,000 RPM, and so turns out it's one of the oldest toys that we know. We found archaeological samples. It's one of the oldest toys civilization. Nobody, nobody had figured out the physics of this object. So we tried to figure out a complex physics behind this. We engineered these things to go up to 125,000 RPM. All of this for, you know, cents. And of course, what it means in real life is now you can take a droplet of blood and you see there are small plastic shims over here. You take Scotch tape. You tape a capillary filled with about 10 microliters of blood, which is this drop, and within 30 seconds it'll separate out to plasma and red blood cells. That's a test for anemia that affects almost 1 billion people.
Steve McLaughlin: You brought a couple other things with you today. I think they might be kind of things that you're working on. May not want to share too much, but I think one thing looks like a regular lighter, like a Bic kind of butane lighter. What, what's, and so for listeners, I'm looking at a butane lighter.
Dr. Bhamla: The reason I brought this and this is when I give talks as well. Often the question is, how do you come up with a solution to a problem? How do you come up? Is it just random chance or is there a system?
As an educator, for me it's important to be able to teach people, right? I would like everybody to be able to develop these frugal tools. So here’s a problem that a bunch of high schoolers brought to me from rural Georgia in a public school, and they said they needed an electroperator. These kids are part of an international team called the International Genetically Engineered Machines competition every year at MIT, and they just actually won gold.
And so the idea they wanted to do was they wanted to do synthetic biology and take E. coli, a bacteria, and modify it to make it into a sensor to make it as a test for, say, cholera. Challenge is to actually modify, and today you'll hear about techniques such as CRISPR and other techniques. A key central part of this is electroporation, which is… What you do is you apply a huge voltage to a cell and you depolarize the membrane for a very short fraction of a time, maybe 5 milliseconds. And what you do is you open up holes in the bacteria wall in the membrane, so you can now inject synthetic gargle or plasmids that you want. Now it could be RNA, DNA, and you've modified the cell into a synthetic machine, right? The electroperators, it turns out, cost thousands of dollars. These high schools, even in Georgia. So there's a lesson here that even for frugal science we go all the way across the world. Turns out even in our backyard for kids to be able to do things.
So they asked me if I could purchase an electroperator for them. The gist is, of its functioning, is you have to apply a voltage. The voltage you need is about 2,000 volts, 5 milliseconds.
The answer is right here. And so I was just giving a talk, and I'm saying I'm—so right now, this is what I'm doing. I'm just clicking on the lighter. And you can hear this noise. So every time you're on Fourth of July when you're doing your barbecue, you play with this.
[lighter flicking]
Dr. Bhamla: So again, the key is in the noise. So what's happening over here? It turns out. So what I just did was press the button and you hear the stick in fact and your stub and you actually twist the knob. You hear this tick tick tick tick tick. You see a spark on your barbecue lighter. And you can buy this at any gas station.
Turns out that every time you click on this spring-based mechanism, there is a tiny metallic hammer that's going, and we've calculated this, at 30,000 meters per second squared. Hits a tiny piezoelectric crystal and generates about 2,200 volts in less than five milliseconds due to piezoelectricity, the phenomena that the Curie brothers discovered. And so this lighter is pretty robust and remarkable and repeatable feat of engineering that generates these voltages. Of course, the butane is just to kind of use that spark to light a flame. And so we take this piece of engineering. You can buy it at any gas station, 30 cents. In fact my high schoolers cannot buy lighters, so I had to buy them. And then we understood the physics of this object, and it turns out that that voltage is perfectly poised. So we take E. coli and then we put GFP molecules in there and lo and behold, we do our test and the E. coli turned green and you can do a lot of different things. You can put it on mammalian cells, in yeast cells.
And so here we have something for 20 cents. You now have an electroperator. And we're writing this paper up.
And so what I'm proud is that the first author is a high schooler, second author is an undergrad in my lab, third author is a high school science teacher, and the fourth author is, you know, an early career researcher.
Steve McLaughlin: We've talked about two things: the Paperfuge. We've talked about the—
Dr. Bhamla: Electropen. We're calling it the Electropen, because the—I didn't bring the actual device, but the kids have it. It looks just like a pen. You just hit a button and you put it on the glass slide and you can inject it.
Steve McLaughlin: And I think you have. I think you've got another one that maybe, maybe you want to highlight something around a hearing aid?
Dr. Bhamla: The 30,000 feet view and the need that we are trying to address is that currently, for the audience who’s listening, you may use hearing aids or know parents or grandparents who use hearing aids. And it turns out these things cost a lot of money. They are thousands of dollars. In the US, in August 2017, the Senate passed a bill to tell the FDA to figure out how to bring hearing aids as over-the-counter devices, because as you grow older, just like you need reading glasses that you can go to buy at a CVS, you should be able to buy hearing aids. And so we were motivated by this need for affordable hearing aids, and not just in the US. Turns out this is a global issue and thousands of dollars for a device that you would ask. It's just amplifying sound or is it? And asking what is, you know, a low cost device that we could build?
My undergraduate student who's leading this, he's a chemical engineer and was born with hearing impairment in both his hears. And so that's one of the things that's beautiful. Sometimes we solve these problems because we resonate with the challenges that lack of one sense that we might have, whether vision or hearing, and he is obsessed and driven by this problem and so the device we've made is a low-cost hearing aid, and it falls within our frugal science philosophy and we're at the stage of demonstrating that, you know, indeed we could have really ultra-low-cost device and starting to ask the question, what is the bare minimum device that we can have that is effective? And those are hard questions to investigate. That's the current project that we're working on.
Steve McLaughlin: OK. I'm going to change directions a little bit and just talk about your background and how you got attracted to engineering, how you develop the curiosity you have, and the skill sets around the problems, and what kind of advice you give to young people based on your experiences, your pathway.
Dr. Bhamla: So this is the part of the conversation in which I wish I had a story where I could say as a kid I was fascinated by science and engineering and I played with, built, you know, rockets and stuff.
Unfortunately, my story is I wasn't much into science. I was an average kid, and I in fact sometimes was below average. I still have report sheets that shows I got a zero in math and I failed and I struggled. I think a lot of it has to do with having the right set of mentors who got me excited. I think the best way I can describe is it allowed me to come into myself and be myself and look at—they shared their view, philosophy of how to approach problems and what they found exciting in science.
And so I have this collection of things I get excited by, whether it's organisms or hearing aids. So it's a combination of mentors and just being yourself and finding someplace where you're allowed to be that and do the best you can.
Steve McLaughlin: What makes you an uncommon engineer?
Dr. Bhamla: I think what makes me an uncommon engineer… There are two parts to this. One is the tangible part is to develop these products that scale, you know, less than a dollar, direct impact. The second more indirect, which helps me sleep at night and I feel satisfaction as an educator, is I show these devices, and often with my—whether it's a high school kid or a medical person out in the field without any education—often they see this and they say, “Huh, I could have done that.”
And to me, that's what the engineering skill set is, is to—because we’re all problem-solvers. You’re a problem-solver, Steve. If things break, you will fix them in your house, in your car, in your research lab, in your life. And almost all of us are and that ability as an engineer to kind of empower people both with devices but use that device as a way to kind of let them realize that the things they are putting on themselves are just self-imposed limits. And I don't know. That, to me, is the way we will solve these grand challenges. If we empower the next generation of students and people in the communities who know the problems the best and who feel that hey, we can do this, I think over the next couple of decades they're going to be able to best solve these problems.
Steve McLaughlin: And your voice is a huge dose of optimism, and I share that same optimism. I think the thing that we're lucky to have you here at Georgia Tech is that inside it you're saying all the ways we've been thinking before clearly aren't working, right? We have all of these challenges and problems, and so we have—and that's the wonderful thing about being at a university is that we have that freedom to really think—our job is to think differently and to really think to be uncommon and to try to come at problems with the different way, because a lot of the approaches we're taking to solve solving problems just simply are working.
So thanks for being here. Thanks for the approach that you're taking, and hopefully there'll be as many students as we can connect to that really creative way of thinking. So we really, really appreciate you being here, Saad.
Dr. Bhamla: Thank you. Thank you, Steve.
Steve McLaughlin: For now, that's all for The Uncommon Engineer. I'm Steve McLoughlin. Thanks for listening.
[jazz music]
[“Ramblin' Wreck from Georgia Tech”]
Geekout
Audio & Captions
Transcript
[music]
Narrator: I'm Steve McLaughlin, dean of the Georgia Tech College of Engineering, and this is The Uncommon Engineer.
[radio modulating to various frequencies]
Radio: Hi, 1-800—
Radio: We live in an age—
[jazz music]
Steve McLaughlin: Sounds incredibly complex. It sounds like—
[radio broadcast]
Steve McLaughlin: I’m really geeking out here.
[radio broadcast]
[jazz music]
[radio modulating to various frequencies]
[jazz music]
Professor: And we showed that whether if few spin a few more minutes beyond just a binary test of plasma and red blood cells, because that you're spinning at such high speeds and the density of each component in your blood, red blood cells, white blood cells, you know, proteins, is different. You can actually create this layering and in that, if red blood cells are infected with malaria parasites, that tiny parasite affects its density to the third decimal place. But because we are spinning so quickly, we can actually create a strata. And instead of looking for a needle in a haystack, it's right there in bands and you look at it under a microscope and it's right there.
Steve McLaughlin: For the listeners, it really is. It's a piece of paper that, you know, a circular piece of paper that, I think, has like a plastic clip on the edge of that. As you pull the pipe apart, the paper spins.
Professor: So now Steve is actually playing with the Paperfuge.
And the more—the reason I did this is because here is an expedience of translating this, because as engineers, we are often used to building things and publishing papers and saying, “Well, somebody else is gonna take it out on the field.”
When you build a device that requires a training of, say, weeks or hours or days… Here, illustrating took—it was so simple, even a 5-year-old can do it, right?
Steve McLaughlin: Even a Dean could do it.
Professor: Even a dean.
[laughter]
Professor: And essentially, that is for when we think about samples and the number of patients that show up at these clinics, thousands of them, and we want to train a lot of clinical health workers with minimal training. And things, you know, you don't want to.
This enables us to now—one person could train the other and this is part of the translation and that was what I wanted to show, that the effort required, you know, how hard was it to pull this off? Often, it's with these things that reason I bring these things and I kind of tell people to use it. You could read the paper and then you use it and you kind of understand. There is much more in the engineering that goes into enables real-world application rather than just—we can all write papers, but that other gap is where a lot of technology fails and we try our level best.
So when we published this paper, you know, we had 15 pages of supplementary information just predicting and describing the mathematics, and one key thing that we realized is the reason these soft, flexible, pliable strings enable us—this answer is the question of, what is the most efficient way to convert, you know, translation, the movements of your muscles into rotational movements.
It turns out that using rigid components such as in salad spinners or your egg beaters, you're using gearing mechanisms. What we are exploiting is actually the flexibility of strings. And the key thing, the theory, the physics that I use was actually used from DNA super-coiling, because what happens if you watch very closely is that the strings actually go from just twisting to actually forming super-coiled structures that displace out of plane. And that enables these strings to act as springs that store energy.
Steve McLaughlin: To get to 125,000 RPM.
Professor: Exactly, and so that was kind of the a-ha moment that—look, physics are complex and you figure out an interesting way to actuate things, and now people have built on this to new applications in generating electricity, because and you know—and that's the cool thing. It's so simple that—and cheap that you can think of 10 different things you could do with it.
[jazz music]
Professor: I feel very lucky, because Georgia Tech is one of those places that attracts people who really want to solve problems and are very-open minded and curious, which is a key recipe, because curiosity, you know, curiosity drives a lot of our stuff and the problems we choose. And so the students come from backgrounds, so from physics, from bioengineering, chemical engineering, of course, and I also find that the—so far in this short duration that we've been here, some of the students have multiple degrees, so minor in say computer science or a minor in art.
[jazz music]
Professor: This is the other part of my lab, which works in organismic biophysics and where we look at organisms and specifically from the lens of extreme biology, asking the question of, what is a fast animal in nature? So if I asked you that, what is the first animal that comes to—
Steve McLaughlin: I’d take the cheetah or something like a—
Professor: Yeah, so that's kind of the common notion of what we think of as fast as cheetahs and diving birds, for example, and hummingbirds. And it turns out—and this is a growing community of biologists and engineers and for the last decade or so using high-speed imaging, which is, you know, modern technology is being able to look at it, look at different organisms, and found that there are organisms such as a mantis shrimp, a crab jaw, and even jellyfish, pneumaticus from jellyfish that achieve accelerations of almost 10 million meter per second square in nanoseconds. Compare that to a cheetah’s acceleration, which is about 10 meters per second squared, or Hussein Bolt for that matter.
Kind of forces your notion to ask what the heck is a fast movement and fast in what? Velocity? In terms of acceleration? And so it turns out acceleration is actually an interesting question to ask.
So we wrote a paper earlier in the year, analyzing hundreds of these engineering systems and biological systems, to find universal physics and to show why as engineers we haven't built these tiny robots that, you know, Feynman and other—Asimov had—you know—we've been thinking about this for what? 60 years? There’s plenty of room at the bottom. But where's the robots that, you know, you swallow as a pill and go through your bloodstream?
And so the paper outlines that there are some challenges. That's why we haven't built these tiny robots, and of course, it's a very complex thing. But some of them relate to power delivery. How do you store energy and deliver it at small scales? And that's kind of a challenge in building autonomous robots.
And so we look at it from a biological system to discover how these systems accelerate very quickly, because if you just take a derivative of that as just power delivery, and so that's kind of interesting, and you realize that biology has all kinds of unusual motors beyond muscles to release and store energy and materials from very complicated materials, such as the resilin and mantis shrimp in crustaceans’ bodies do, you know, geometric latches and springs.
And so I was a year ago or two years ago actually, you know, in the rainforest in the Amazonian rainforest in Peru where we were doing some fieldwork testing our low-cost devices. And I took a day off, because as a child I'd always wanted to go to the Amazonian rainforest, you know.
And so we’re there on the last day. One of the guides showed me this tiny spider. He said, “Look at this spider. It moves really fast!”
And indeed, it did! I couldn't capture it on my iPhone, because it not only goes up to 200 frames per second in slow motion. And so I came back thinking, like, you know, and I Googled and you know. The best thing as a young scientist is when you Google and you see, holy smokes, there's no mention in the scientific literature. It's like in 2018, when do you ever get to Google an insect and see there's nothing known about this.
So while we took a—I hired a postdoc, and this year we went back to the Amazon rainforest now with high-speed cameras and we discovered this spider that we are calling the “slingshot spider.” And we're writing this paper, so I don't want to say it too much, but the gist of this is that it achieves accelerations of 600 meters per second square. And so we're kind of going piece by piece, trying to answer the question of what the heck is it doing? How is it doing this? Why is it doing it? And we have pretty good answers for some of these hypotheses. And to the best of our knowledge, this is the fastest movement by an arachnid that we've kind of recorded movements of in less than millionths of a second.
And so there are two points here:
One is you said why should an engineer care? And you know, the engineering part is, you know, silk is one of those biomaterials that has, if you think of a biomaterial that has inspired engineers and material scientists more, silk is up there. And here we've realized that silk is, you know, you think of it as strong as Kevlar. What we realized is this silk in this particular case in this rainforest spider is not just strong in Young's modulus but actually is very, very elastic and acts as a very complicated three-dimensional network of springs, similar to what you would think about Leonardo da Vinci's catapults but you know in 3D.
So that's one, and the second is kind of a… We do a lot of outreach in Lima with a lot of high schools. And you know, when I ask the same question over there, these kids will tell me the fast movements are cheetahs. You know, they don't have any cheetahs in Peru, but the kids are reading textbooks from France and from Europe. And I try to illustrate to them, look, your backyard is full of incredible biodiverse, you know, living creatures, both insects, arachnids, and all kinds of things.
Here is a tool, now this low-cost tool that you can use to actually explore your own biodiversity and discover things, because you know, now you can. In 2018 you still can and now you have things you can put in your backpack. And so we share with them, and the kids you know they…
That's kind of the second part of this. We kind of encourage them that we lose so many organisms every day that go extinct. We just don't know.
And to me what's beautiful about this is we all care about encouraging hands-on science, but often hands-on science, implicit in that is you give them, you know, tools. Information is free on Google, right? But how do you give them the hardware?
And here we are turning these kids into problem-solvers, because you want to do synthetic biology and do CRISPR, but you need to build your own tools, and that's kind of a… to me is this is our second device that we're kind of writing up now and sharing this. So to the many different lessons here and teaching, you know, students to be problem solvers also. But also participating in the whole circle of science is going from a brainstorming idea, from a whiteboard, to building thing and to writing a paper, because this high schooler now knows much more about, you know, sharing his science through the language that you and I do as papers and enables them to participate, right? And encourages the other high schoolers to be problem-solvers as well.
The a-ha moment with all these things is one night we do one experiment. It is like holy smokes, it works. But then we spend years trying to figure out why the heck does it work? Because these kids will bring me data that looks on point, right? 2,200 voltage with a standard deviation of only like 100 volts. I don't believe this. Are you doing your measurements right?
And so we had to understand the physics turns out that it's independent of user, because a lighter is a lighter irrespective of who fires it? A kid or a grandmother.
That's because the forces depend on a spring and the spring constant inside. Until you actually understand the mechanics, it turns out who studies the mechanics of lighters? We had to, so that's part of our favor—
The reason for this is important because the next step we're taking this is into gene-based vaccine delivery, and you need to control the voltage for mammalian cells and that's different because these cells are not, you know, 10 microns versus bacteria which is a micron. You need a different voltage field. The spring content allows us to change that, and that's where the science comes. We understand why the heck it's work—
And then the same theme applies where I just showed you that we translate it. So now we share these designs and devices now and people in the school, high schoolers in Taiwan tested it across Georgia to different schools. And so people reproduce our results, and it only takes a week, because, you know, we don't—we ship them one, but we just give them the 3D printer files and these kids download it.
The demonstration over here is that, of course, our science is reproducible across the ocean. But there is also a need. I initially misjudged how… not easy but how much hunger there is and how much the high schoolers can do synthetic biology but are often restricted by scientific tools, so the frugal science is again a workhorse tool that we are developing that we're not going to tell them what's living sensor to make, but we're going to give them the tools that get them to unleash their imagination and creativity.
[echoing] Even a dean could do it.
Professor: Typically, as engineers often we love to think, what if you had infinite resources? What if you had $1 million? $1 billion? And that's kind of where I come from in the Bay Area. It's like, if I give you, you know, $1 million what can you do? Can you change the world?
And we like to take the exact opposite approach. And you know, the analogy my colleague was drawing, what would Gandhi do? Or how do you think, if you had nothing? What would you do with bare minimum?
And it turns out, for some of the problems we get about, that is the reality of the situation for a lot of people and they might not have access to infrastructure, electricity, basic things that you and I would say, “Oh, just plug it into the wall,” but there's no plugging in. There's no batteries. What do you do then? And that's kind of the constraint we deal with.
[“Ramblin' Wreck from Georgia Tech”]